What is a forest?
Â
Although ‘forest’ is a commonly used word, it is not easy to clearly define what it is, as it largely depends on the national context. There are more than 800 different definitions for a forest around the world! But the most recognized approach to identify a forest, used also by the Food and Agriculture Organization, defines forest using indicators such as: 1) trees of minimum height of 5 m, 2) at least 10% for crown cover (proportion of the ground shaded by the crowns of trees) and 3) a minimum forest area size of 0.5 hectares. By this definition, there are just under four billion hectares of forests on Earth, covering about 30% of the total land area. About half of the world’s forest areas are in three countries: Russia, Canada, and Brazil.
Â
Types of forests
Â
Forests are usually classified in terms of the predominant tree species – broadleaf, coniferous (needle-leaved), or mixed – and their leaf longevity (whether they are evergreen or deciduous). The main biomes of the world are presented in Fig. 2.3.1 and include the following forest types:
Â
- Boreal forests (taiga) are generally evergreen and
- Temperate forests include broadleaf deciduous forests, evergreen coniferous forests and a mix of both types. Warm temperate zones support broadleaf evergreen forests.
- Mediterranean forests are generally composed of evergreen broadleaf and sclerophyll Sclerophyll means ‘hard-leaved’ in Greek, as such trees usually have small, dark leaves covered with a waxy outer layer to retain moisture in the dry summer months. Coniferous forests also occur in this zone.
- Tropical and sub-tropical forests include moist broadleaf forests, dry broadleaf forests, and coniferous forests.
Figure 2.3.1 The main biomes of the world
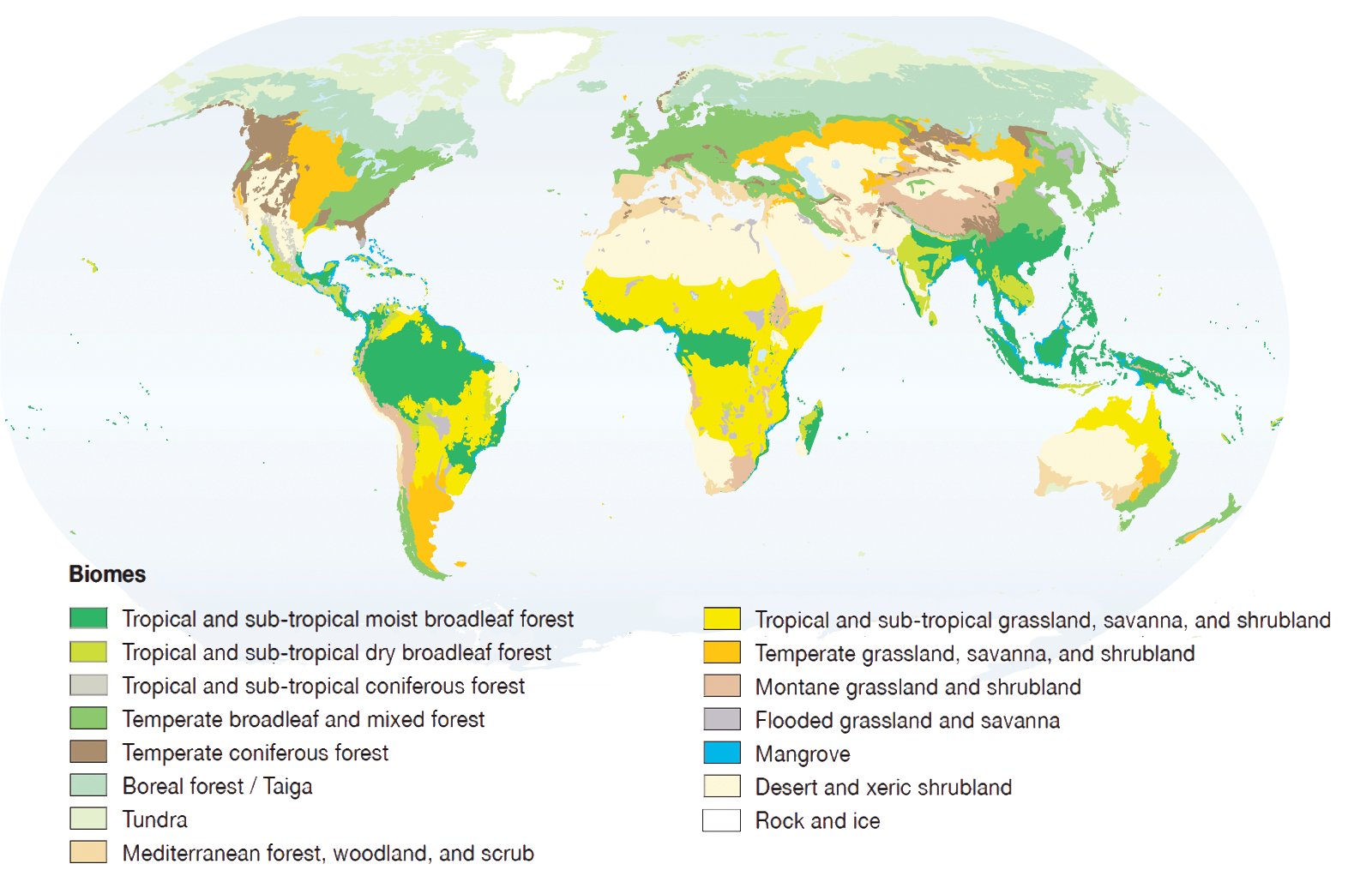
Why are forests dependent on the climate?
Â
The life of the forest and its geographic distribution depend on climatic conditions, especially air temperature and the amount of precipitation. Only in some places on our planet is the climate suitable for forests to grow. For example, the location of the northern-most forest line depends on the average annual air temperature. Where it becomes too cold, boreal forest is replaced by tundra. However, air temperature, especially on the plains, changes only gradually. So, the border of the forest and tundra becomes a transition zone, where areas of both tundra and forest are found. This transition zone is called forest-tundra (Fig. 2.3.2).
Â
The southern line of temperate forests, where forests give way to grassland (steppe) and semi- desert, is determined by rainfall. In hot conditions, plants and trees are constantly losing moisture from their leaves to keep cool. If rainfall in the summer is scant, there is not much moisture in the soil, and trees have difficulty drawing it upwards as high as their crown. Because the air is warm and precipitation is limited, low herbaceous plants are at an advantage and the landscape becomes steppe.
Â
Relief, soil quality, water bodies and human activity are also important in determining forest cover. The share of forest diminishes in regions where much of the land has been put to work in the economy.
Figure 2.3.2 Forest-tundra
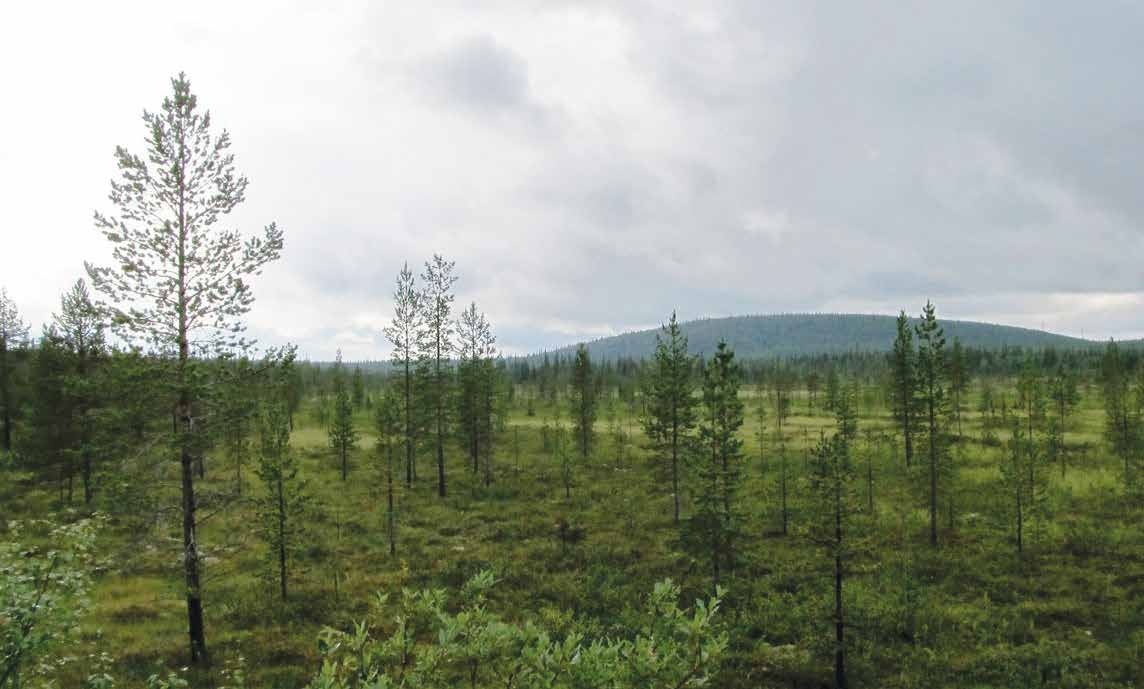
Forests of temperate and sub-arctic climate zones
Â
Boreal forests (taiga) are dominated by coniferous tree species: pine, spruce, larch, fir and cedar. It is interesting that such forests in Europe and Western Siberia consist mainly of pine and spruce, while in Central and Eastern Siberia they are mainly larch trees. This is due to the permafrost in the vast Siberian territories, which make these areas particularly well suited to larch.
Â
Differences in temperature conditions (average summer temperatures, times of formation and melt of the snow cover) justify subdivision of the taiga zone into northern, middle, and southern taiga. Mature trees in the forests of the northern taiga do not grow high, reaching 10–20 m, while in the southern taiga they may be as high as 50 m (Fig. 2.3.3). The middle taiga is intermediate between the northern and southern, not only geographically, but also in terms of the average height of trees, which grow to 20-25 m.
Â
The area to the south of the taiga is occupied by a zone of temperate deciduous forests (Fig. 2.3.4). It is dominated by various species of oak, hornbeam, and elm. Such trees are commonly known as deciduous hardwoods (because their wood is relatively hard). To the south of the deciduous forests in Eastern Europe and Central Asia, the grassland (also called ‘steppe’) begins, and the transition zone is called forest-steppe. However, there is no deciduous forest zone in western Siberia and the central regions of North America (Fig. 2.3.1), where taiga gives way immediately to grassland. This is due to the regions’ continental climate: rainfall is low, so the ground is very dry and deciduous forests, which need a lot of moisture, cannot grow.
Figure 2.3.3 Southern taiga
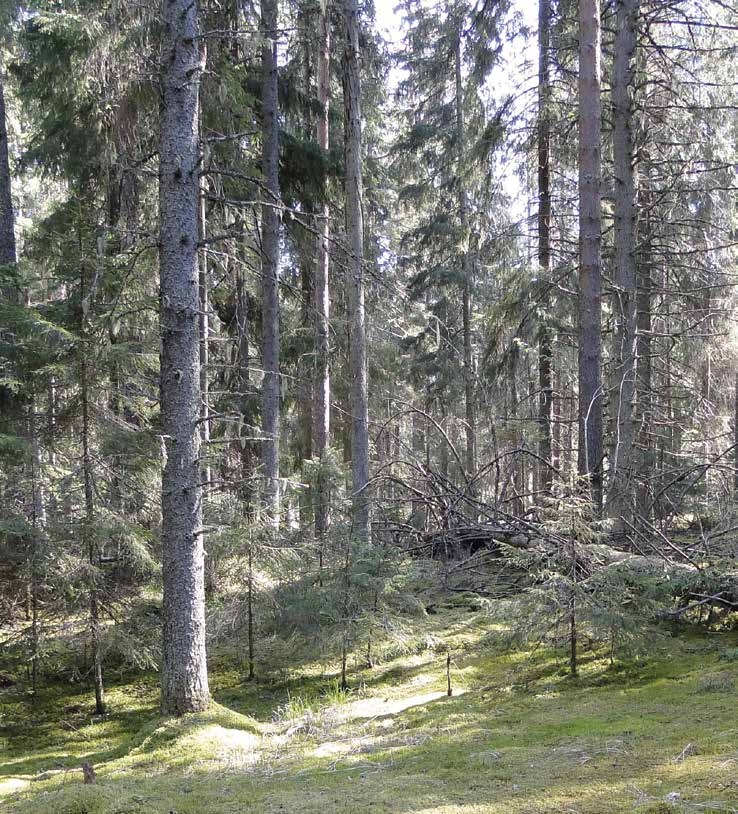
Figure 2.3.4 Deciduous forest
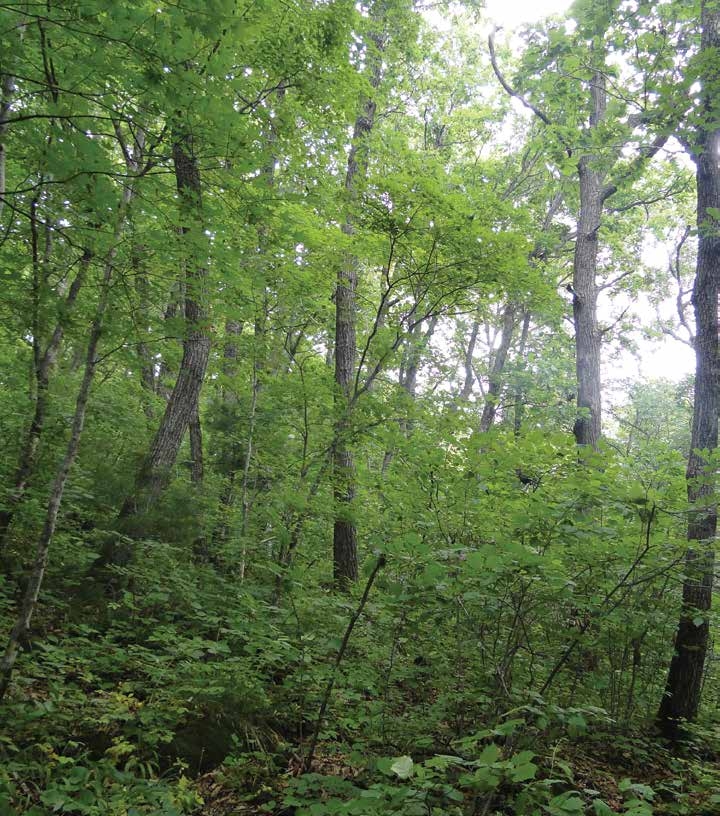
Does climate change taking place today have an impact on forests?
Â
Are forests affected by the current warming of the climate? Yes, they are!
Â
Climate change will have many widely varying effects on trees and the composition of tree species within forest ecosystems. As the climate continues to change, trees must either adapt to new conditions or migrate to more suitable locations.
Â
Changes associated with global warming are particularly apparent on the northern boundary of boreal forests. In polar regions, trees and shrubs rise are moving higher up the mountain slopes, gradually usurping the place of mountain tundra (Fig. 2.3.5). The upper boundary of larch trees on mountain slopes of the Polar Urals (Russia) has moved upwards by 35–40 m in the last 80 to 90 years and by 50–80 m in some regions. Shrubs are now growing more than 50m higher up on slopes in the Khibiny Mountains on the Kola Peninsula (Russia), and an intensive growth of shrubs, particularly willows, has been observed in Scandinavian tundra zones.
Figure 2.3.5 Change of vegetation cover in the Polar Urals (Russia)
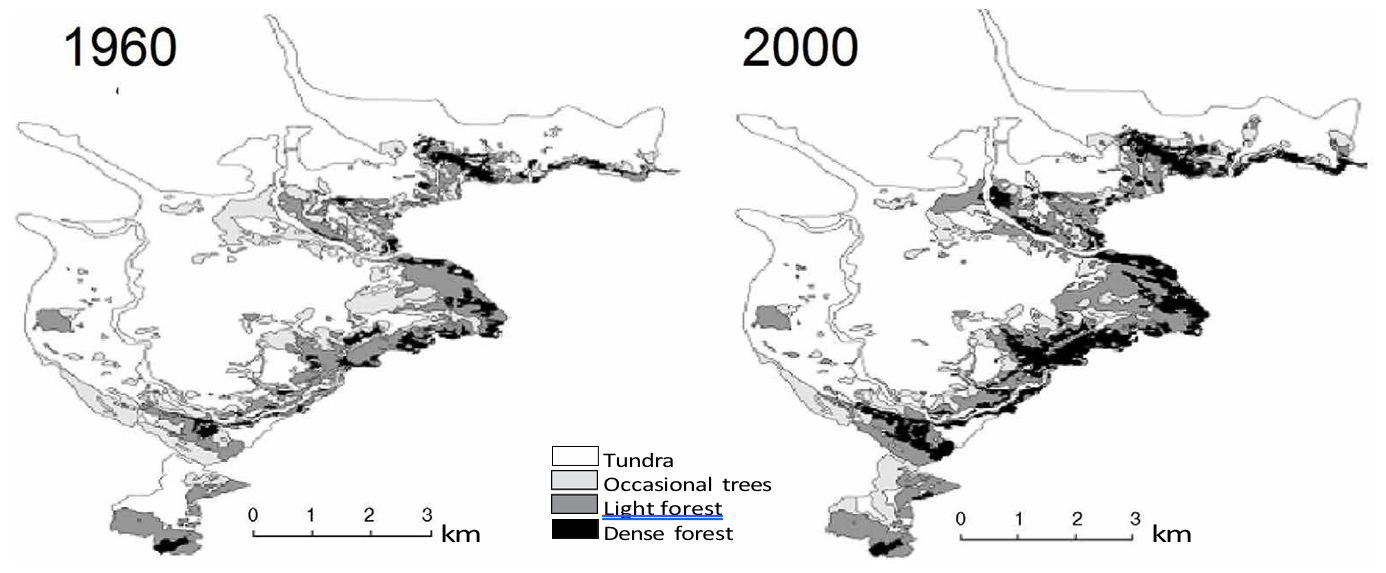
Heat is driving trees up mountains in South America
Â
Trees and shrubs in mountainous regions of South America are fleeing unbearable heat on the plains by moving up the slopes of the mountains where the air is cooler, making it possible for them to survive. In the Andes, trees are moving up mountains by an average of 2.5–3.5 m each year. This is a considerable feat for plants, which cannot move except by reproducing. But climate change is happening so quickly in the Andes that trees need to climb more than six metres each year to remain in a comfortable temperature.
Â
Of the 38 plant species being monitored by scientists, the Scheffleris species is migrating the fastest of all: it rises about 30 m higher each year. The fig tree is unlikely to survive in these regions by 2100 if global temperatures rise by 4°C as it is moving higher at a rate of only 1.5 m per year. Climate models suggest that more than 50% of tropical plant species could become extinct.
The southern boundary of the temperate forests is also changing. Oak forests are gradually disappearing in the forest-steppe and steppe zones due mainly to summer droughts. In the region around Lake Baikal, by contrast, pine forests are advancing into steppe ecosystems, due to increased precipitation. So, the southern forest line is shifting because of changes in moisture levels rather than an increase of temperature.
Â
The areas of Russian forests that are occupied by tree species have changed in recent decades and scientists believe that this is largely due to climate warming. For example, oak forests have diminished in southern regions, but are gaining ground further north, on the border between deciduous forests and the northern taiga.
Â
Spruce forest (Fig. 2.3.6) is in retreat in nearly all parts of Russia. The root system of spruce trees is close to the surface, which makes the tree highly sensitive to increase in the frequency and duration of droughts. At the same time, many regions of Russia are seeing an increase in birch forests. This phenomenon is well known to forestry specialists: it is because, after a fire or the felling of coniferous trees, birch and other small deciduous species initially appear in their place, and some time is needed before new coniferous trees appear and begin to displace birch, aspen and alder. However, in recent decades, this last stage has not been occurring: conifers seem unable to regain ground lost to birch forest.
Figure 2.3.6 Spruce forest
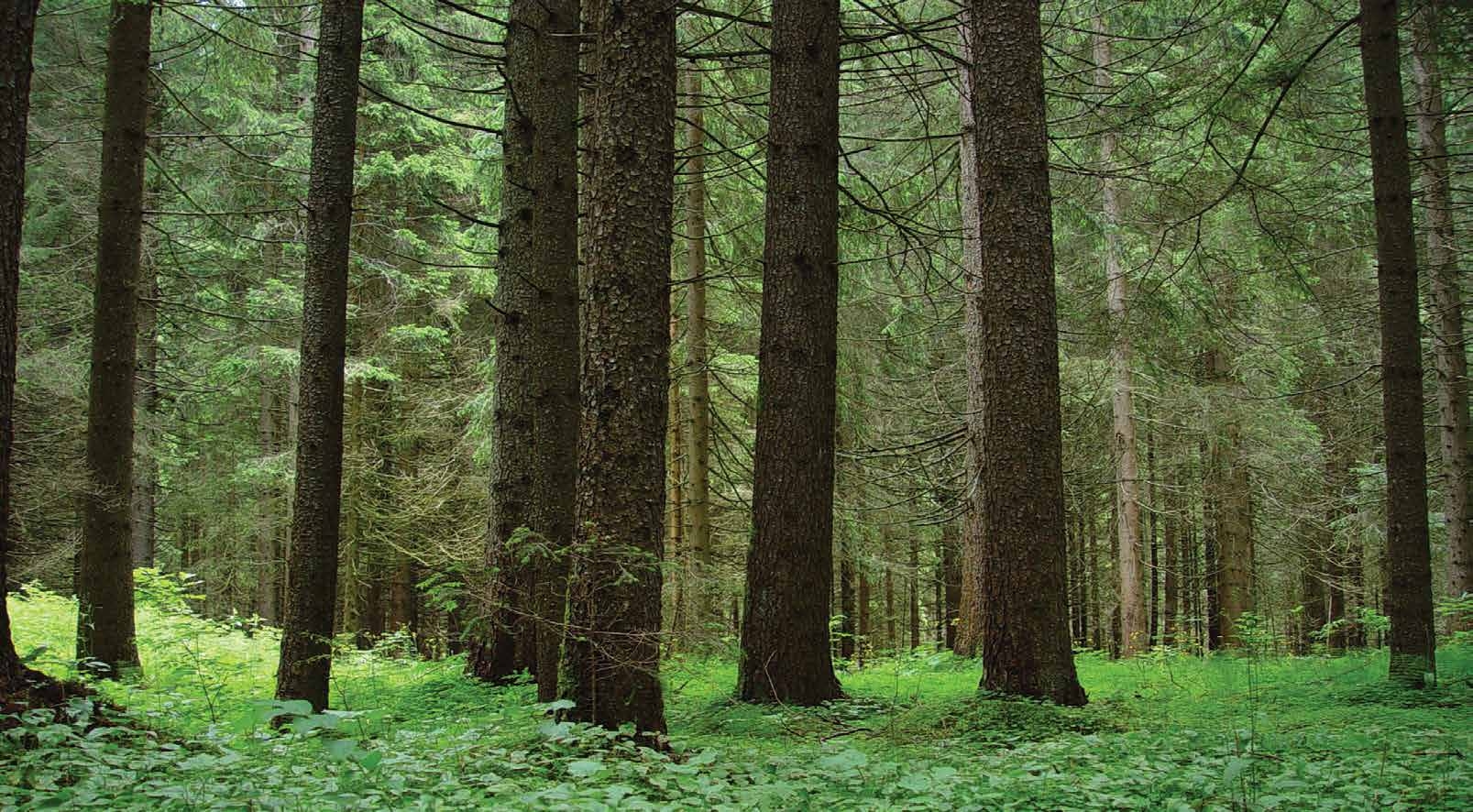
Most forecasts predict that forests in northern parts of Eurasia and North America will be more affected by global warming than forests elsewhere in the world, as their northern and southern boundaries are displaced. A rise in temperature of 2°C will cause an increase in the total area of forest cover in Europe of taiga to advance into the tundra zone. However, an increase in temperature of 4°C will cause the southern forest boundary to retreat northward and this effect will be greater than the northward advance into the tundra zone (Fig. 2.3.7).
Figure 2.3.7 Forecast changes in forest cover in Europe by 2100, assuming global warming of 2°C and 4°C.
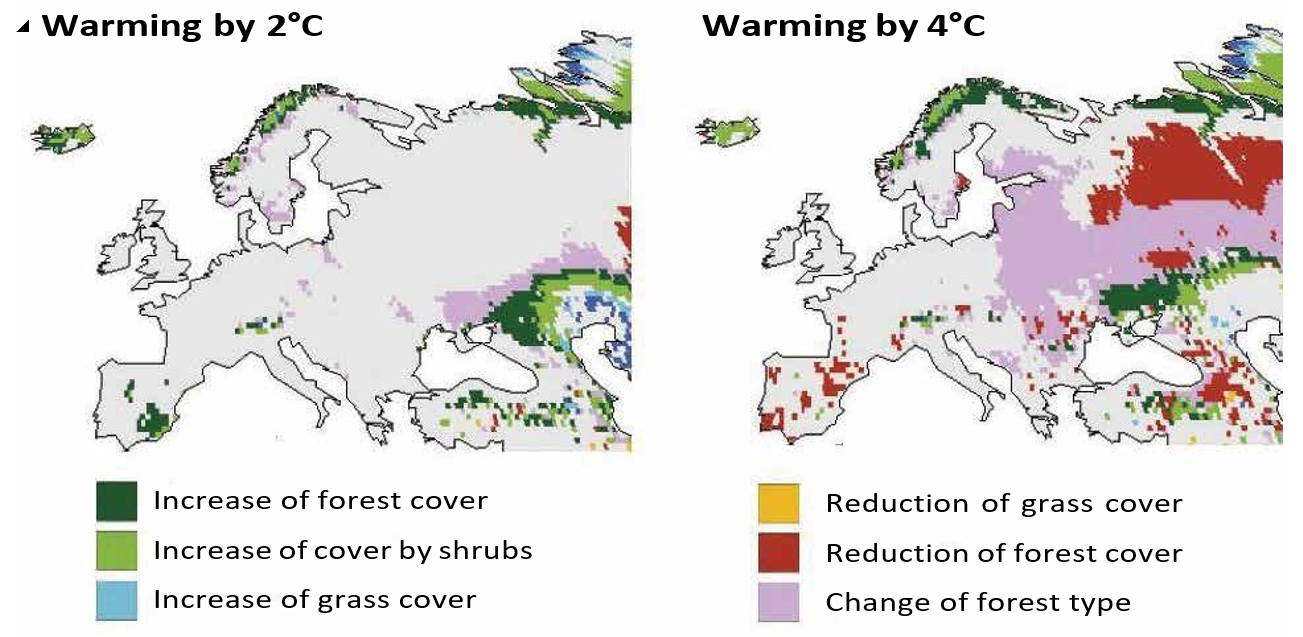
Deforestation caused by climate change will affect almost all Eastern Europe and Western Siberia. This is a worrying forecast, showing how serious a phenomenon the disappearance of forests may be in a worst-case scenario for global warming.
Â
Destruction of forests by fires, pests, and extreme weather
Â
Another major threat to forests associated with climate change comes from large-scale fires and plagues of pests caused by hot weather in summer. Heat and other extreme weather events are often directly responsible for the destruction of forests.
Â
Forest fires are usually started accidentally by people. But they can take hold only in certain conditions, namely, when the weather remains hot and dry for days or weeks. The forest floor of dead leaves, pine needles, small branches, mosses, lichens, and grasses growing under the forest canopy then becomes dry and catches light easily, and the fire can spread over large areas. This is called a ground fire.
Â
When fire spreads in coniferous forests it often reaches the crowns of trees. Pine needles and small branches of fir and pine trees contain large amounts of resinous substances, so living trees can easily catch fire. A crown fire is the most dangerous and destructive and can lead to the complete loss of an area of forest (Fig. 2.3.8).
Â
Fires cause great damage to the forest: many trees perish, their growth is stunted, the variety of trees in the forest becomes poorer, and the spread of harmful insects and pests is made easier. As the climate changes, the risk of forest fires increases because higher temperatures dry out woody materials more quickly. The warm season of the year, during which fires can occur, also becomes longer.
Figure 2.3.8 Forest fire
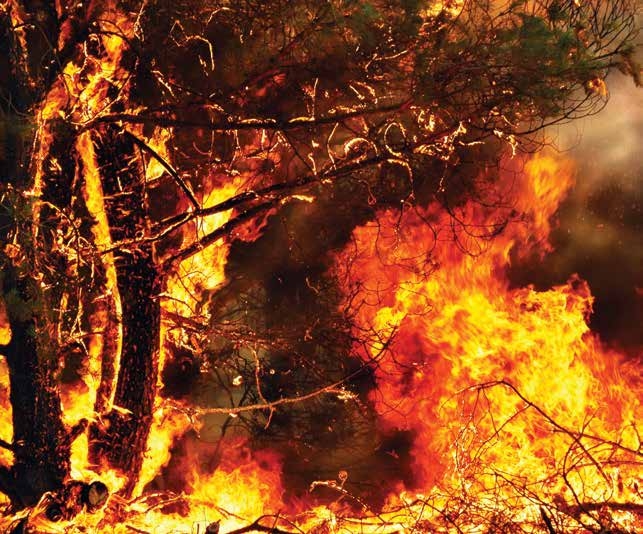
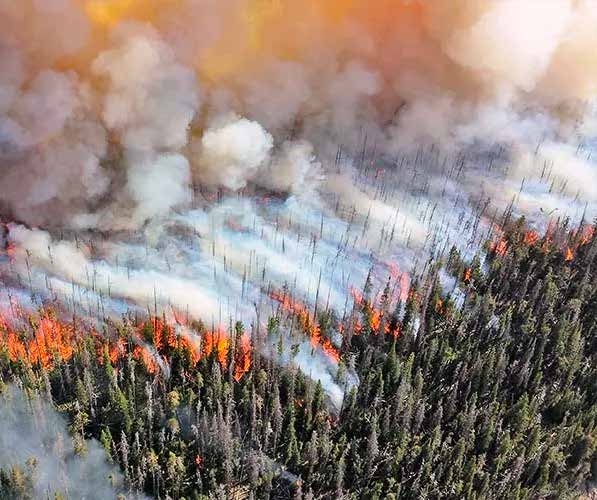
The unusually hot summers of 2010 and 2012 in the central part of European Russia weakened conifers that are accustomed to very different conditions. Such weakened trees are easy prey for many species of insects that live under the bark. In years when temperature and humidity levels are normal the population numbers of such insects are controlled by other species (insect and bird predators). But if the population of bark beetles becomes too large, large tracts of forest may perish! The dried-up trees first lose their needles, and then their bark. Various fungi that attack wood go to work on the roots, which are eventually unable to support the trees. Strong winds can then blow the trees down, one after another (Fig. 2.3.9). First, birds and then squirrels desert the forest where they can no longer make their harvest of cones. Martens then move elsewhere in search of better hunting. The green forest floor, made up of lilies of the valley and wood sorrel, which flourished thanks to the protection from direct sunlight afforded by the trees, is replaced by thickets of reed grass, raspberry bushes, and nettles. In short, the entire range of species is changed.
Â
All is not lost: the displaced species can return. As happens after a serious fire, a forest of spruce will fully restore itself after one or two hundred years. But only provided that such forest has remained intact elsewhere with all its inhabitants, and abnormal fluctuations of temperature do not recur.
More recently, in September 2021, fires have destroyed more than 18.16 million hectares of Russian forest, setting an absolute record since the country began monitoring forest fires using satellites in 2001. The previous record was set in 2012, when fires covered 18.11 million hectares of forest.
Â
Other extreme weather events – hurricane-force winds and tornadoes – can destroy forests as effectively as drought, by blowing down trees (Fig. 2.3.10). Heavy rains can also do much damage by washing away the soil or killing trees through prolonged waterlogging. Heavy falls of wet snow and the large-scale formation of ice also harm trees, and heavy showers of hail damage bark on the branches, weakening and causing them to dry out.
Figure 2.3.9 Fallen spruce after the drought of 2010
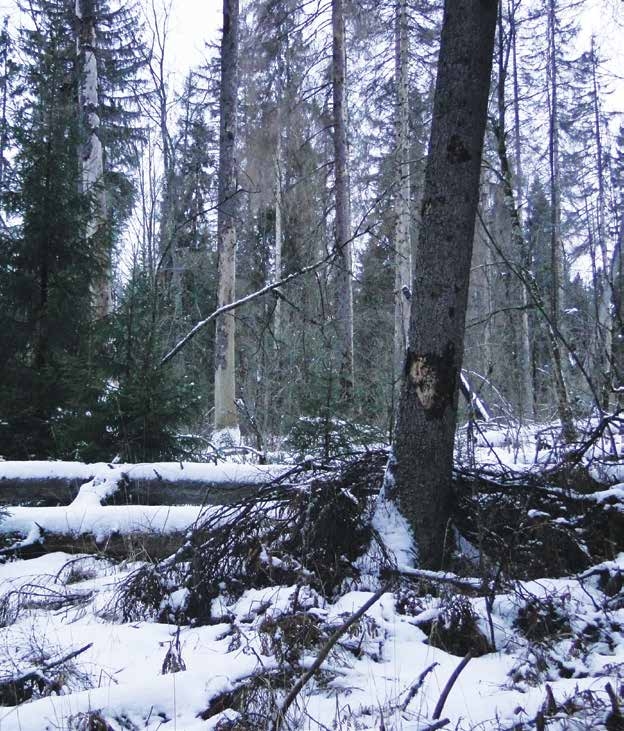
Figure 2.3.10 The aftermath of hurricane-force winds in Kostroma region
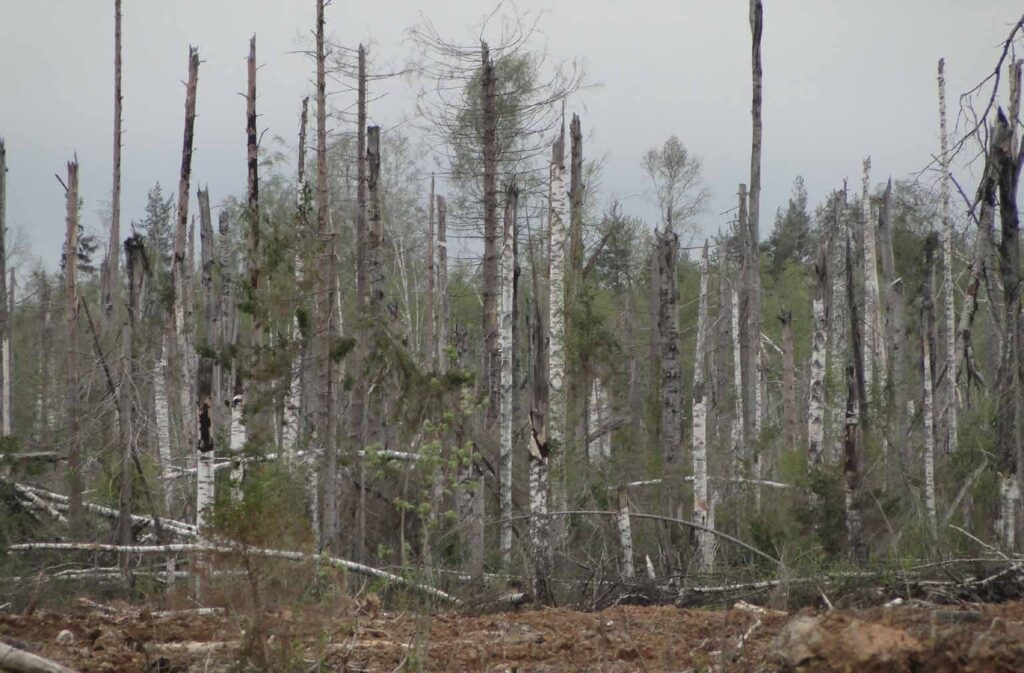
The history of glaciation, along with current scientific evidence and forecasts, show that forests and other natural ecosystems can adapt to the most varied climatic conditions. But this adaptation is mainly related to migration – that is, to changes in the boundaries of natural areas and vegetation types. During periods of glaciation, forests survived in a relatively small area, and large expanses of Eurasia were covered by tundra and tundra-steppe. When the climate grew warmer, forest regained its status as the dominant vegetation type. But warming of the climate today is happening too quickly, threatening not gradual, but catastrophic change of vegetation types, through the large-scale drying-out of forests with high risk of forest fires.
Â
This makes it highly important not to let global warming reach extremes, and to work toward a gradual stabilization of the climate change on the planet.
Â
How do forests affect climate?
Â
We know now how climate and climate change affect forests. But this relationship also holds in the other direction: forests have an impact on climate!
Â
For example, green forest changes the reflection of sunlight by the Earth’s surface, so it affects the amount of heat absorbed by Earth. The difference in temperature between forested areas and areas without forest are especially noticeable in the winter. The sun’s rays are reflected from the treeless, snow-covered plains, but the dark spaces of boreal forests reflect less and absorb more of the sun’s light.
Â
Forests help to retain moisture in the soil and affects evaporation, making the regional climate milder and wetter.
Â
Snow cover remains longer in the forest, lessening sharp changes of temperature that occur in the springtime and reducing the risk of spring flooding by rivers.
Â
But what makes forests particularly important for climate is the carbon cycle. Carbon dioxide released into the atmosphere by the burning of fossil fuels is the main cause of the global warming occurring today. Forests play the vital role of absorbing carbon dioxide from the atmosphere and retaining carbon in the form of various organic substances.
Â
You may know that green plants absorb carbon dioxide and produce oxygen. This process is called photosynthesis, and it is powered by the energy of sunlight. Forests represent a dense concentration of green plants (trees, shrubs and grass) and are therefore thought to be vital for enriching our planet’s atmosphere with oxygen. You often hear the term ‘green lungs of the planet’ used on TV and in newspapers to describe forests. Absorption of carbon dioxide and emission of oxygen are the two sides of the single process of photosynthesis, so you would think that forests must remove carbon dioxide from the atmosphere. But it is not that simple. To understand the process of exchange of carbon dioxide between the forest and the atmosphere, we need to understand how the forest stores carbon, the element that joins with oxygen to form carbon dioxide. All organic substances contain carbon. For example, nearly half of the weight of dry wood is carbon.
What is a carbon pool?
Â
Any part of the ecosystem that contains significant amounts of organic matter is a store of carbon. Scientists call such stores ‘carbon pools’. Carbon pools can do both, take or release carbon. There are four main carbon pools in the forest ecosystem: 1) phytomass or biomass (the weight of living plants); 2) dead wood; 3) litterfall (dead leaves and branches on the forest floor), 4) organic matter in the soil. In many countries there is a much smaller fifth pool, called harvested wood products.
Â
The phytomass pool consists of living plants: the trunks, branches, roots, leaves and needles of trees and shrubs, the leaves and roots of grass and moss (Fig. 2.3.11). As a rule, tree trunks account for most of the phytomass, but moss is also a major part of it in northern boreal and marshy pine forests.
Â
The dead wood pool consists of dead trees and roots. The death of trees in the forest is called ‘dieback’ and it occurs naturally as the growing trees compete for sunlight. The smaller trees are left in shadow by the larger trees and gradually wither because they do not receive enough light for photosynthesis. This is why young forest is much thicker than old forest. But attrition can also occur in various other situations: it can be caused by forest fires, droughts, forest pests, and man-made pollution. In forests that are affected in one or several of these ways, the carbon pool in dead wood may exceed the pool in living wood.
Â
Litterfall is made up of relatively small fragments of organic matter lying on the soil surface (Fig. 2.3.13). It consists mainly of dry leaves and pine needles, small dry twigs, flower petals, cones and other fragments that have fallen from living plants. In deciduous forests replenishment of the litterfall pool is most intensive during the autumn leaf fall, while in boreal forest it occurs more evenly through the seasons.
Â
Soil pool in the forest contains significant amounts of carbon. The soil is a mixture of minerals and of organic matter, mainly ‘humus’, which is a dark-coloured substance created by the gradual break-down of plant residues (litterfall, dead wood and dead roots). Carbon accounts for 58% of the make-up of humus, which is a higher share than in phytomass. The darker the soil, the more carbon it contains (Fig. 2.3.14).
Â
The carbon stored in wood products is often referred to as the harvested wood products carbon pool. Some wood products, such as high-quality wood furniture and wood framed buildings, can hold onto carbon longer than if the tree had been left in the forest. Wood products are important for helping bank existing forest carbon while harvesting helps give space for replacement trees to grow.
Â
In boreal forests, phytomass contains 21% of the carbon stock, dead wood 4%, litterfall 3%, and 72% is in the soil. So, in these forests, carbon is concentrated in the soil.
Â
These shares are quite different in tropical forests, where living and dead organic matter accounts for 50% of carbon.
Â
Why is the difference so large? In boreal forests most dead plants are broken down by fungi and bacteria, and this process occurs slowly. It takes many decades for the trunks of large dead trees to disappear. Because of this, the forest accumulates large pools of dead organic matter – dead wood, litterfall and humus in the soil. In tropical forests, a large part of the litterfall and dead wood is consumed by animals, especially termites. This speeds up the rate of decomposition and reduces the contribution of dead organic matter to the total amount of carbon in the ecosystem.
Â
Figure 2.3.11 The wood in tree trunks is the biggest part of the phytomass carbon pool
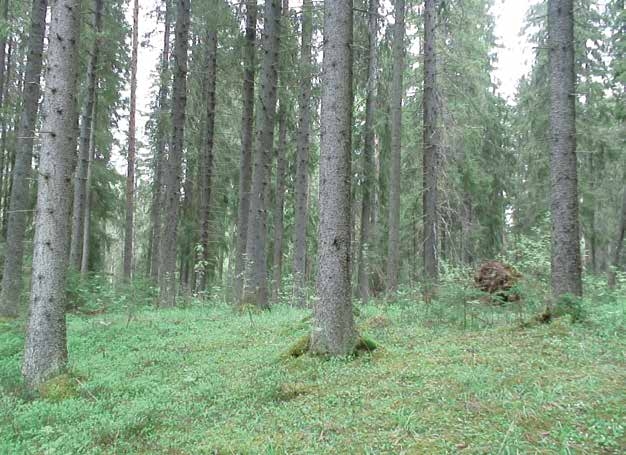
Figure 2.3.12 Dead trees are part of the dead wood carbon pool
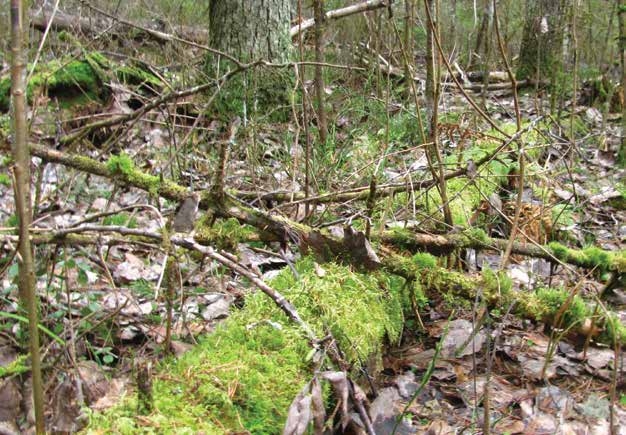
Figure 2.3.13 The litterfall carbon pool is swollen when leaves fall in the autumn
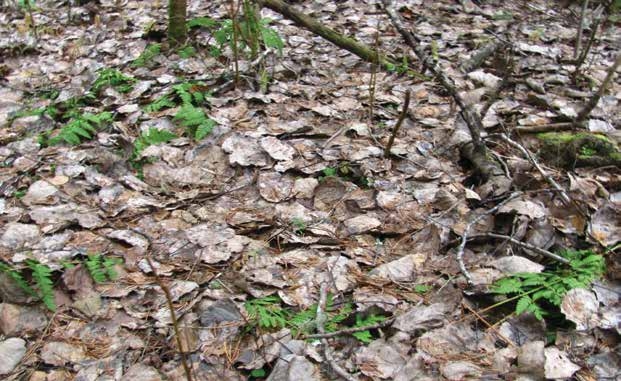
Figure 2.3.14 If soil is dark, it contains a lot of carbon
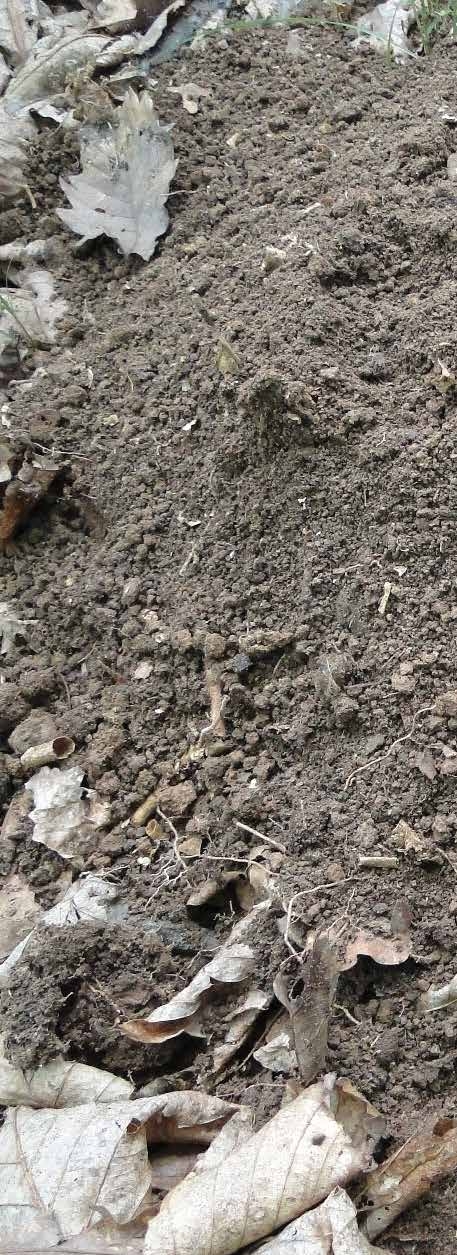
Carbon budget
Â
Now that we know all about carbon pools in the forest ecosystem, let’s see how these pools relate to one another and the atmosphere (Fig. 2.3.15). Scientists call this system of connections a ‘carbon budget’ because it is like the financial budget of a country, a company, or a family, where what comes in (income) must be matched with what goes out (spending).
Â
The only ‘income item’ in the forest ecosystem is photosynthesis. The sum of photosynthesis creates organic sub- stance. The first consumers of this sub- stance are plants themselves: nearly half of it is used by plants when they breathe, releasing carbon back into the atmosphere. The remainder of the substance is called ‘net photosynthesis’: it replenishes the phytomass pool.
Â
Various living organisms that inhabit a forest consume the living substance of plants: caterpillars and insects feeding on leaves; birds and rodents collecting fruits and seeds, and hoofed animals that eat grass and young branches.
Figure 2.3.15 The carbon budget of a forest ecosystem
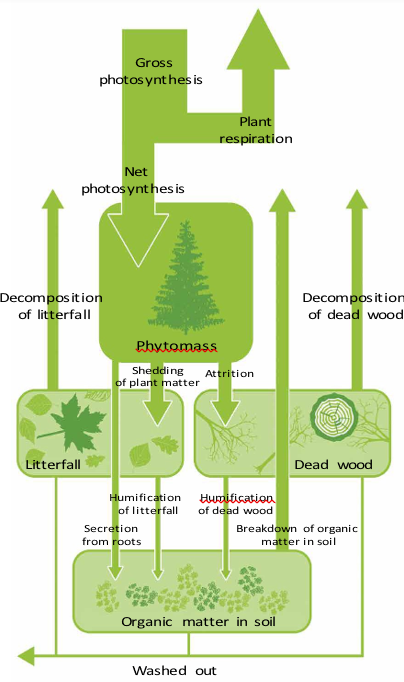
In taiga and temperate forests, a large part of the plant life dies naturally (when a plant withers and dies or when it drops its leaves and twigs) and is then consumed by fungi and bacteria (Fig. 2.3.16). This replenishes the dead wood and litterfall carbon pools.
Â
When fungi and bacteria breathe, the carbon humification of litterfall secretion from roots of organic matter binds with oxygen and returns to the atmosphere as carbon dioxide. This happens as dead wood and litterfall decomposes. A modest part of these pools is transformed into humus and replenishes the soil carbon pool (this process is called humification). Carbon also enters the soil from living plants in the form of organic substances that are secreted by the roots.
Â
Organic matter in the soil is also broken down by fungi and bacteria with the release of carbon dioxide into the atmosphere. A part of the carbon is washed out of the ecosystem by groundwater and surface water: you must have seen autumn leaves being carried away by forest streams.
Â
Forests that contain many mature and old trees absorb the same amount of carbon dioxide from the atmosphere as they release back into it. Carbon pools in such a forest remain constant over time. They are like swimming pools: full to the brim and incapable of taking more, except that they are filled with carbon and not water. But that is not to say that mature forests don’t play a role in regulating the gas composition of the atmosphere. The point is only that they no longer actively absorb and have instead become the keepers of ‘stored’ carbon, i.e., carbon that can no longer contribute to the greenhouse effect.
Â
The carbon budget of young, growing forests is different from that of mature forests. Young forests accumulate carbon, removing it from the atmosphere. This carbon builds up in pools. So, it is only young forests that deserve to be called the ‘green lungs’ of the planet.
Figure 2.3.16 Tinder fungus breaks down dead wood and returns carbon to the atmosphere
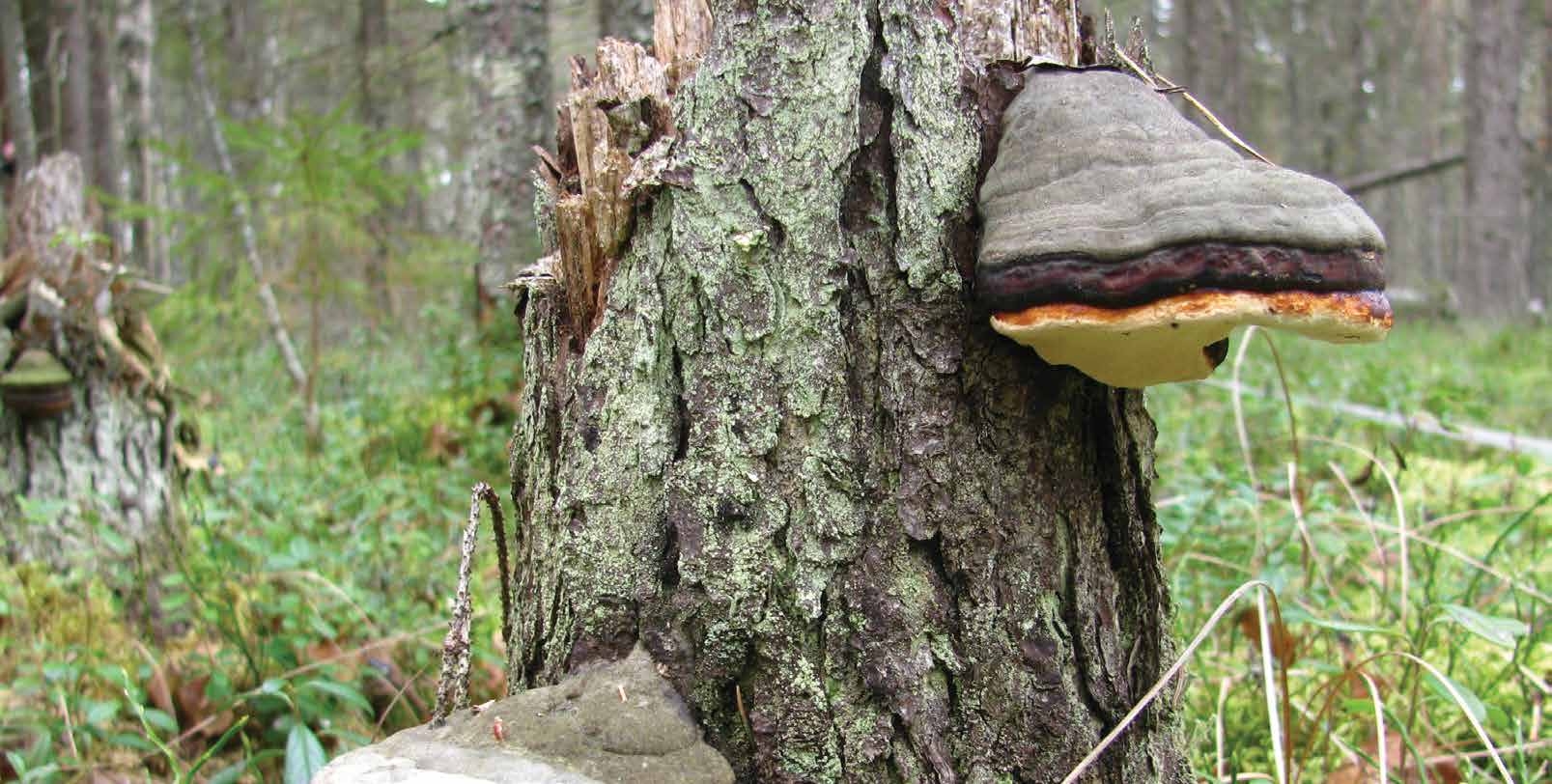
Differences in the impact of forests on the atmosphere
Â
We have seen how young and old forests work differently: young, growing forests absorb carbon dioxide from the atmosphere and can thus partially compensate for emissions of this gas by the combustion of coal, gas, and oil. Mature forests store enormous amounts of carbon in bound form, preventing the formation of carbon dioxide, which would contribute to the greenhouse effect. So, if we want to use forests to prevent climate change, we need to: 1) plant new young forests, where there was no forest before; 2) take good care of existing forests.
Â
In developed countries with advanced economies (the United States, the countries of the European Union, Canada, Russia, and others) there are many young forests that absorb carbon dioxide from the atmosphere and are less at risk of being destroyed for human industry. Many of these countries are also encouraging landowners to plant forests.
Â
Because boreal and temperate forests grow and absorb carbon over many decades and sometimes hundreds of years, carbon is now being accumulated thanks to renewal of the forest in many of the places where forest cover was severely reduced during the industrialization of the last century. The restoration of pine forest along the Canadian Pacific coast is a striking example of this.
Â
At the beginning of the century this territory was covered with huge conifer forests of Douglas Fir and Red Cedar, some of them as high as 80–90 metres. By the middle of the 20th century, these forests had been cut down, and the giant stumps of felled trees more than two metres in diameter can still be seen (Fig. 2.3.17). Since then, strict environmental laws established in Canada have renewed forests in former logging areas.
Figure 2.3.17 This giant tree stump in the forest of western Canada (British Columbia) is evidence of intensive tree harvesting in the first half of the 20th century
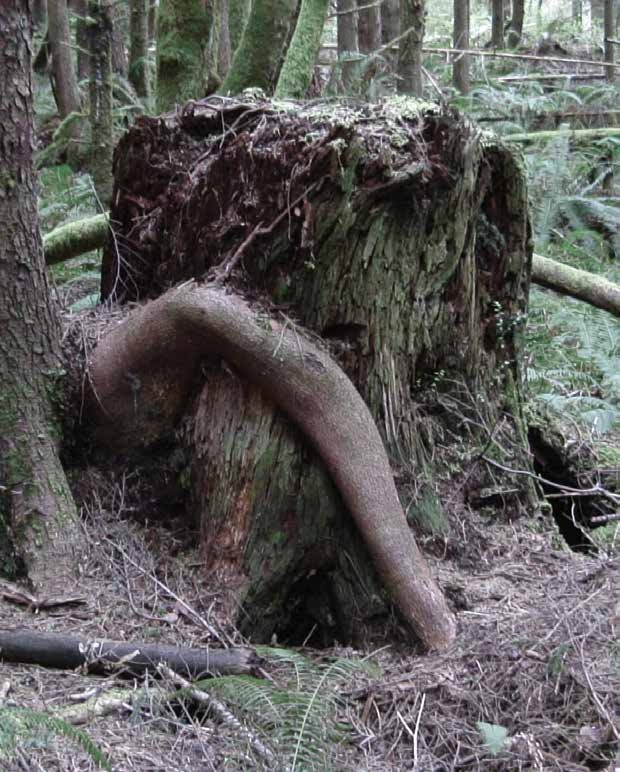
Figure 2.3.18 Loss of forest cover on the island of Bougainville (Papua New Guinea), 1972-2002
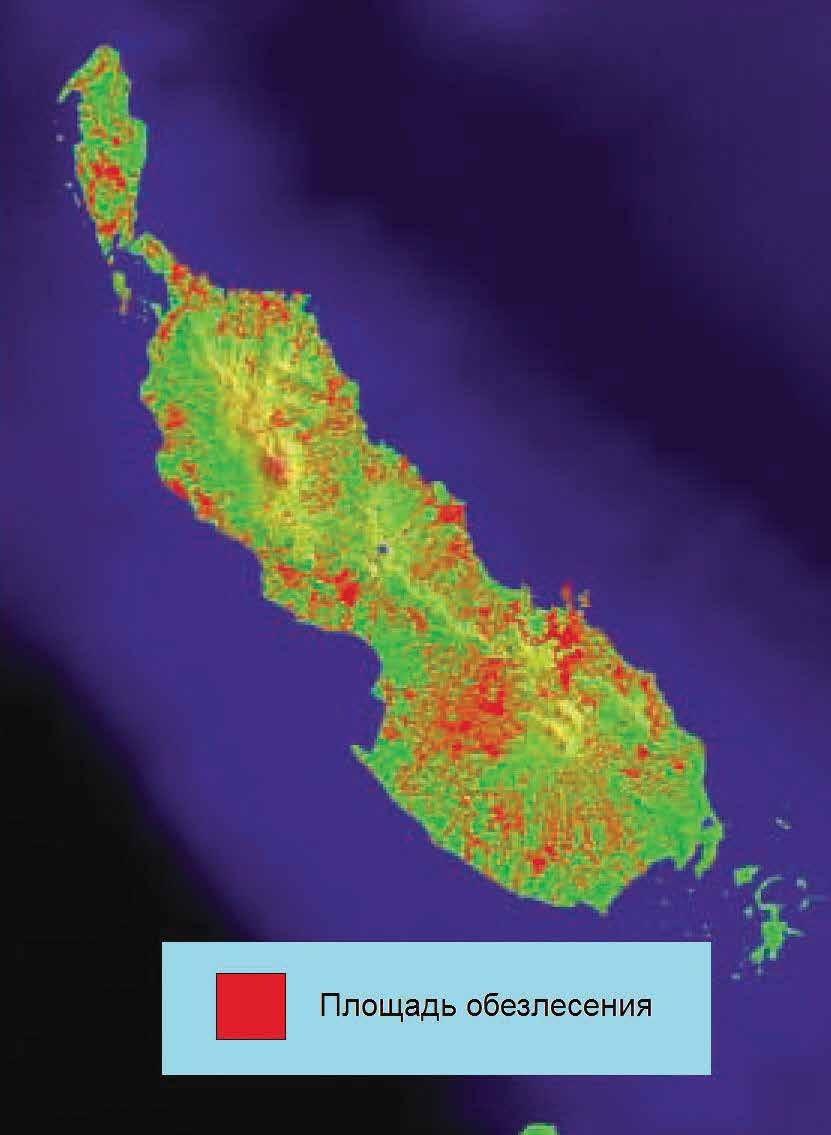
The situation is quite different in developing countries, especially in South America, South- East Asia and Oceania. With growing populations and economies, these countries always need more land for agriculture, factories, cities, towns, and roads. This land comes mainly from the destruction of tropical forests, and new forests that would absorb carbon dioxide are not being planted. A photograph taken in the tropical region of Argentina (Fig. 2.3.19) shows the beginning of the destruction of forest. Forest land previously belonging to the army was transferred to local government control in the early 2000s. Local government gave permission for agricultural development of the land, and the felling of trees began.
Â
Deforestation is occurring very rapidly in some tropical regions. Deforestation in Papua New Guinea is extensive and occurs at a rate of 1.4% of the tropical rainforest being lost annually. Illegal logging is the main cause of deforestation, contributing to 70-80% of all timber exports. Between 1972 and 2002) the country lost more than five million hectares of forests, trailing only Brazil and Indonesia among tropical countries (Fig. 2.3.18). As a result, greenhouse gas emissions from deforestation in Papua New Guinea more than doubled over this 30-year period. Thanks to rainforest conservation efforts, the pace of deforestation has recently slowed to an average 0.5% annually.
Â
About 10% of all the carbon dioxide now being emitted into the atmosphere by human action comes from the destruction of tropical forest. The United Nations programme, Reduced Emissions from Deforestation and Forest Degradation (REDD+), is a step towards a global system to reduce greenhouse gas emissions caused by deforestation in developing countries. Bilateral international projects to preserve tropical forests are being set up, for example agreements between Brazil and Norway, and Australia and Indonesia. Some developing countries, such as China, India, and Costa Rica, have their own programmes to increase the area of their forests. Overall, though, the rapid release of carbon stocks by the destruction of tropical forests remains a major concern.
Figure 2.3.19 A former forest area in Argentina
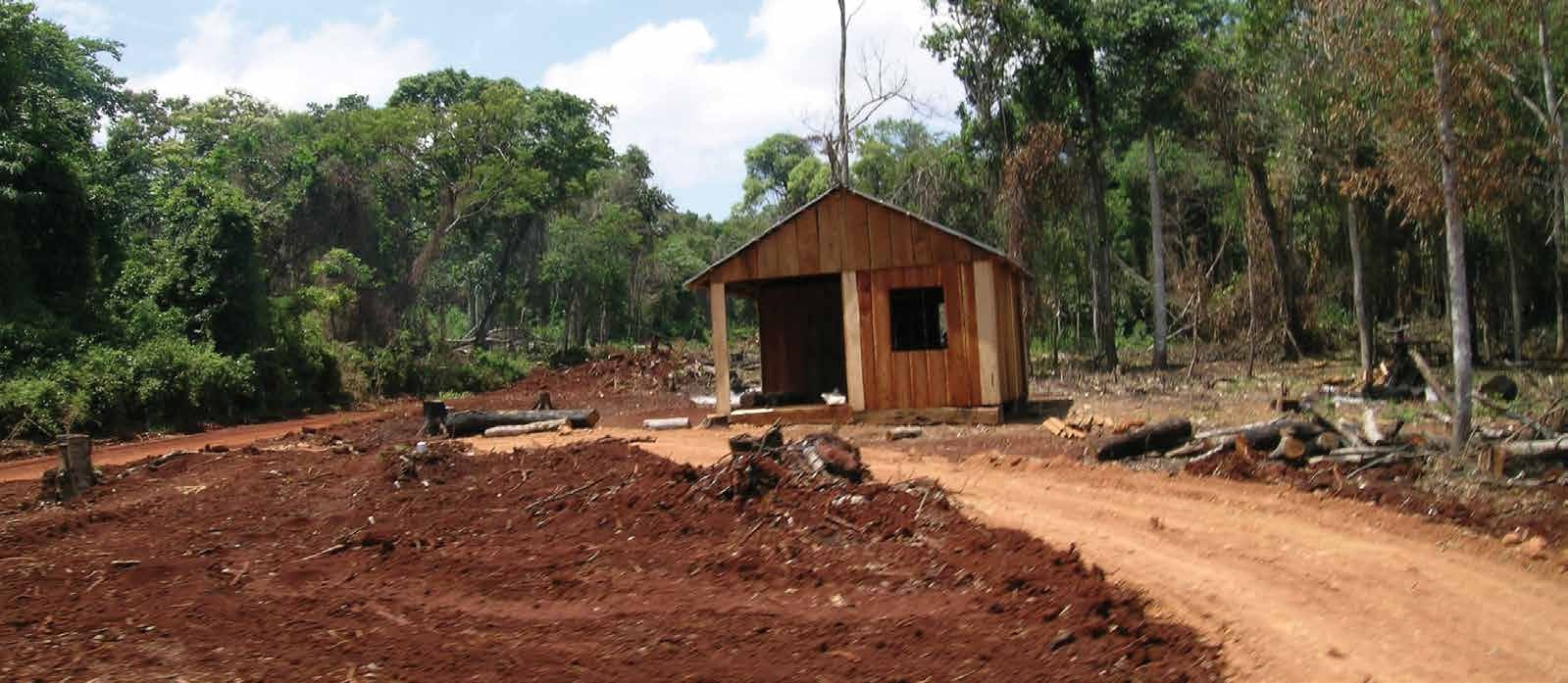
The disappearance of tropical forests
Â
Tropical rainforests are among the most important ecosystems on the planet. Their ecosystem is the richest in species diversity. Tropical forests are a source of timber, food and raw materials for medicines. They also play a pivotal role in regulating the Earth’s climate. The disappearance of tropical forests leads to the loss of fertile topsoil, loss of biodiversity and disruption of the ecological balance over large areas of the planet.
Â
Despite all efforts, however, tropical forests are continuing to disappear rapidly, particularly in South America and Africa. About 3.6 million hectares of forest cover in South America and 3.4 million hectares in Africa have been lost between 2005 and 2010. Today tropical rainforests cover only 5% of the Earth’s surface, compared with 12% 100 years ago. An area of forest larger than all of England (130,000 km2) is being cut down or burnt each year.
Â
In an encouraging development, deforestation has declined and net forest cover increased since 2010. Government initiatives and international moratoria were successful in reducing deforestation in the Amazon between 2004 and 2015, while forest regrowth occurred in Europe, Eurasia, and North America. But keeping deforestation rates low is challenging, given increased deforestation rates in the last four years in the Amazon and elsewhere.
Â
One of the main causes of deforestation is the conversion of forests into agricultural land to feed a growing world population. Rainforests are often replaced by plantations to produce palm oil, soy, cocoa, rubber, and coffee as well as for cattle farming. Uncontrolled mining operations are another threat to tropical rainforests in South America. These are all leading causes of tropical forest loss, destroying some of the most biodiverse places on Earth, home to species including jaguars, sloths, orang-utans, toucans, and lemurs. Such major destruction of forests can be irreversible. If the felling of trees is limited to a small area, then the forest will return after a few years, but if a large area of forest is cut down, it may never reappear: heavy rain will wash away essential nutrients in the soil and the sun will burn the top layer of soil, so that only weeds can grow.
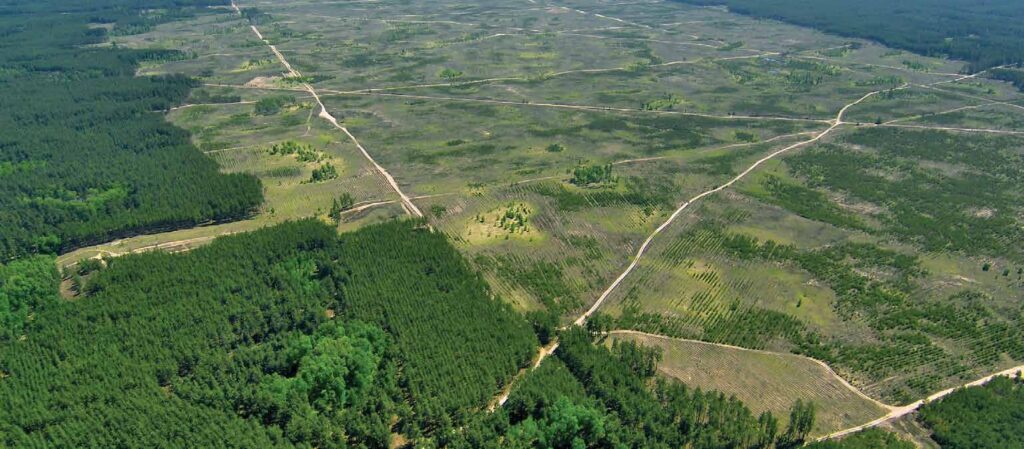
What can be done to save the forests? First, developing countries with large areas of tropical forest (primarily Peru, Ecuador and Indonesia), as well as Brazil, must be encouraged to pursue other economic activities, which do not involve deforestation. Otherwise, the destruction of trees will continue for mining and food production.
Â
Economic incentives and regulations can help to reduce deforestation and prevent forest loss. Brazil had considerable success at reducing deforestation in the Amazon in the late 2000s and early 2010s. Environmental laws, a new Forest Code, improved surveillance of slash-and-burn illegal logging and a soya moratorium in the Amazon were credited with the fall. However, there have since been large spikes in deforestation in the world’s largest rainforest due to lack of law enforcement. Similarly, Indonesia’s recent success in slowing deforestation with a moratorium on palm oil expansion is fragile for the same reasons: the economic incentives to clear forest have not changed.
How to manage the carbon balance of forests and help forests adapt to climate change
Â
The carbon balance of forests depends on many factors, the most important of which are human activity, disasters (forest fires, plagues of pests, etc.) and climate change. The carbon balance of forests can be managed: if the felling of forests for timber and other purposes is reduced, forests will absorb more carbon from the atmosphere.
Â
One project in the far east of Russia aims to halt large- scale logging in the cedar and deciduous forests of the Bikin River Basin, where only local inhabitants will be allowed to cut timber (Fig. 2.3.20). The project encourages residents to develop traditional forms of forest management, including the collection of pine nuts, berries, mushrooms, ferns and herbs.
Â
It is also vital to reduce the damage caused by forest fires, most of which are started by people failing to put out picnic fires, throwing cigarette butts on dry litterfall or lichens, and setting fire to dry grass (Fig. 2.3.21), actions defined as ‘being careless with fire’. We have all been warned to protect the forest from fire, but the warning acquires a new urgency in the face of climate change. If you can teach your friends not to burn grass or set fire to summer fluff, and to carefully extinguish the campfire after a family outing in the forest, you will be doing your part to prevent climate change.
Figure 2.3.20 Cedar-deciduous forest in the Bikin River Basin
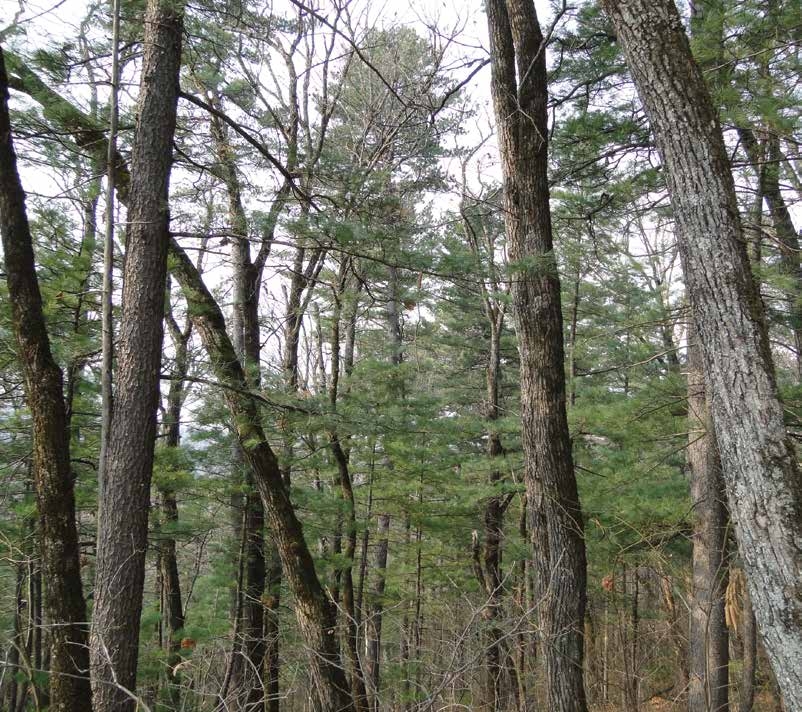
Figure 2.3.21 Dry grass burning, set alight by people
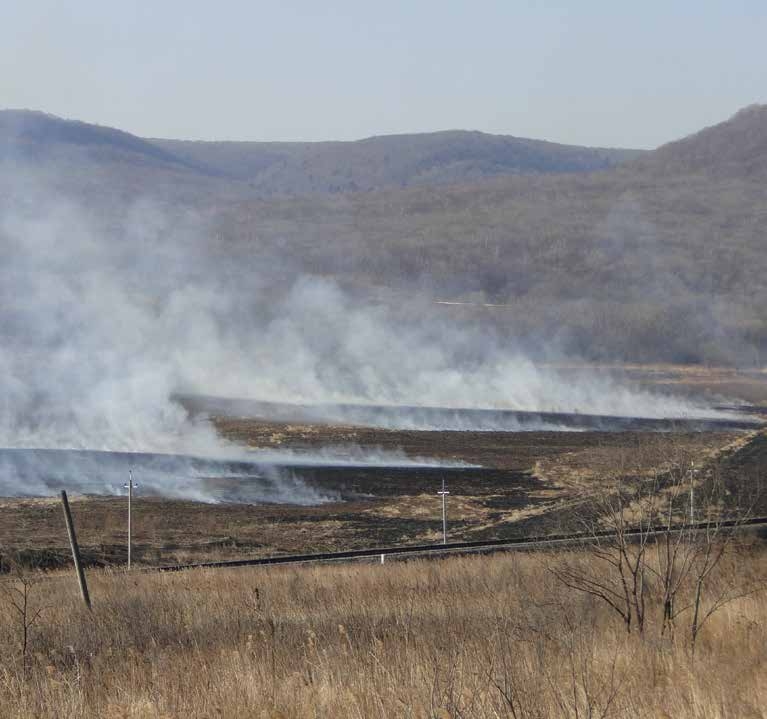
In general, scientists distinguish between adaptation for natural and for managed forests. Adaptation for natural forests includes conservation, protection, and restoration measures. In managed forests, adaptation options include sustainable forest management, diversifying and adjusting tree species compositions to build resilience, and managing increased risks from pests and diseases and wildfires. Forest management is a popular strategy to cope with drought, reduce fire risk, and maintain biodiverse landscapes and rural jobs. Restoring natural forests and drained peatlands and improving the sustainability of managed forests enhances the resilience of carbon stocks and sinks.
Â
The IPCC AR6 notes that successful adaptation strategies for forests in Europe and Russia include altering the composition of tree species to enhance forest resilience. Diversifying tree species and increasing conservation areas reduce vulnerability to pests and pathogens, strengthen resistance to natural disturbances, and may increase carbon sequestration, biodiversity, and water quality. Active management approaches can limit the impact of fires on forest productivity. These include reforestation, fuel reduction management, prescribed burning, changing from conifers to deciduous, less flammable species, recreating mixed forests and agroforestry.
QUESTIONS
1
What is taiga or boreal forest?
2
What species of tree is dominant in Eastern Siberian taiga and why?
3
How has the border of the forest-tundra shifted in recent decades and why?
4
If temperatures rise by 4°C before the end of this century, how will they affect forests?
5
How do human activities affect forests?
6
What are the major carbon pools in the forest ecosystem?
7
Can plants breathe?
8
Which organisms break down dead plant residues?
9
Can mature and old forests remove excess carbon dioxide from the atmosphere?
10
Why are tropical forests losing their carbon stocks?
11
What kind of climate adaptation measures, mentioned in this and other chapters, are recommended to address climate impacts on European and Russian forests?
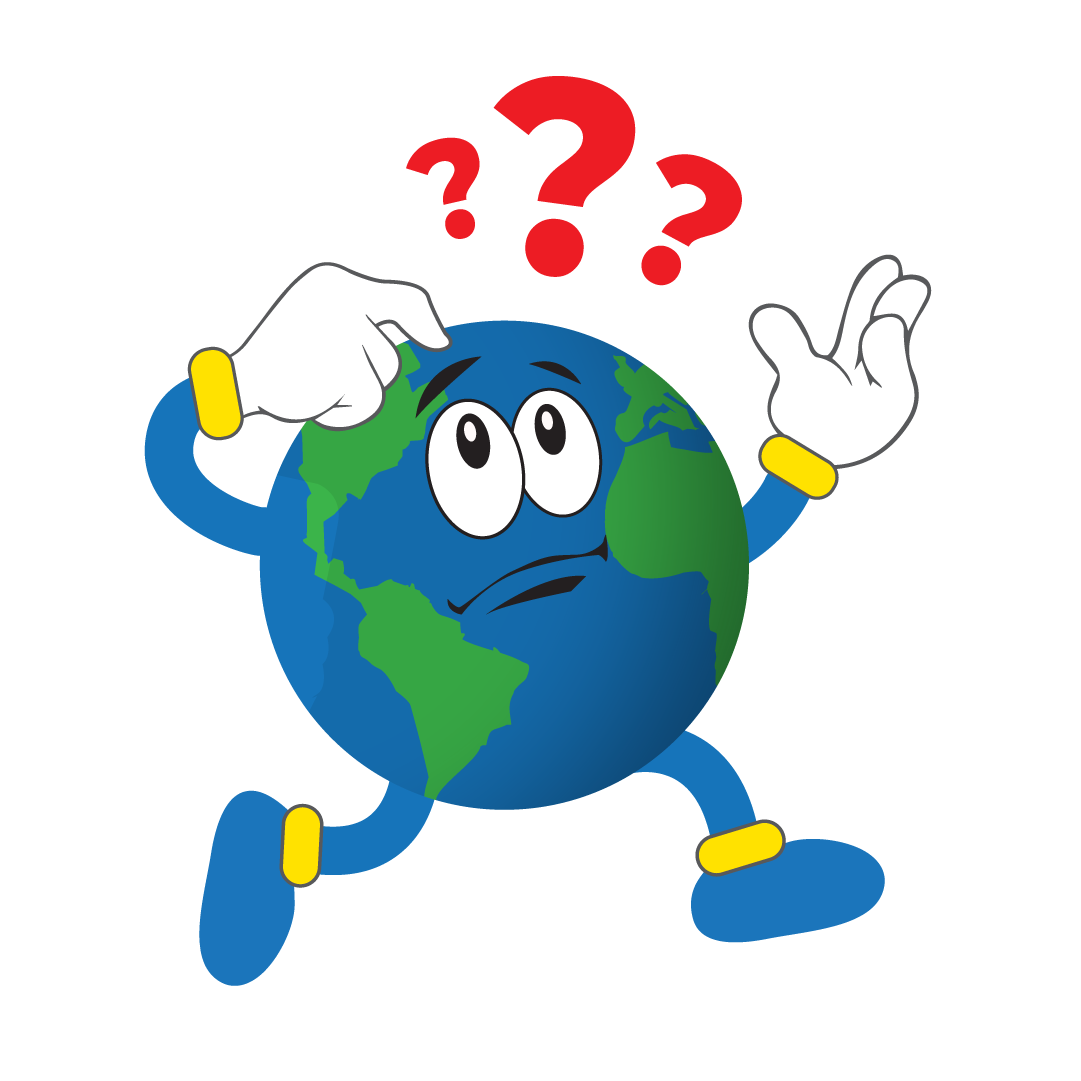
TASKS
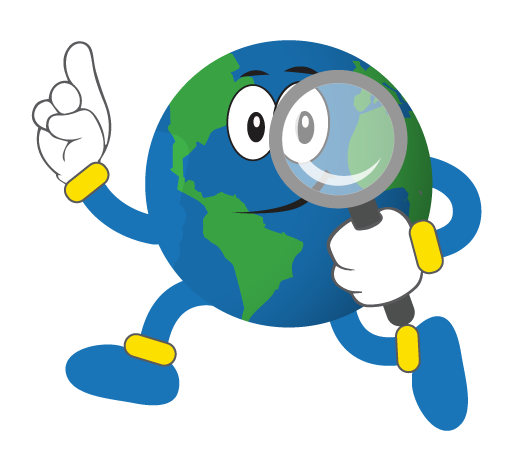
1
Experiment
Objective: To find out which trees and shrubs are most sensitive to warming. Materials: branches of trees (before leaves appear), vases with water.
Â
The procedure: The experiment is carried out a few weeks before snow starts to melt in your region. Cut a few branches from various trees and shrubs (birch, elm, willow, poplar, maple). Put them in vases with water and observe them regularly. Take note of how the buds grow, when they open, and how the leaves grow. Also measure how buds develop on the trees themselves. After leaves have appeared on trees, make a chart to plot the growth in the size of the buds and the leaves indoors and outdoors. Find out which three species are more sensitive to a warmer environment (which of them react faster to warmth).
2
Experiment
Objective: To find out which tree species contain more carbon in their wood. Materials: Pieces of various types of wood (oak, spruce, birch, aspen and others), a ruler, scales.
Â
The procedure: Measure each piece of wood to calculate its volume (multiply the length by the width by the height) and weigh it. Divide the weight of each piece by its volume to find out the weight in grams of a piece of wood with sides of one centimetre. Divide the result by two to get the weight of the carbon in the piece of wood. Discuss the result and decide which tree species has the greater carbon pool. You can then judge which species is best to plant to reduce the greenhouse effect.
3
Experiment
Objective: To compare the amount of oxygen and carbon dioxide emitted by plants in the light and in the dark.
Â
Materials: Two large glass containers with air-tight lids and containing water (about a third of the volume of each container), cuttings of plants with large leaves, a splint, matches.
Â
The procedure: Place a plant cutting in each container and seal it. Put one container in a warm, bright place, and cover the other with a cloth that keeps out the light. After one or two days use a lighted splint to see in which of the containers the flame burns brighter: do this immediately after removing the cover, until the gas in the container has been diffused. Notice the bright flash of flame on the splint you put into the ‘light’ container immediately after removing the cover and notice how the flame dimmed when you put the splint into the ‘dark’ container. Now you know that a plant produces more oxygen than carbon dioxide when it is in the light, but more carbon dioxide than oxygen when it is in the dark.
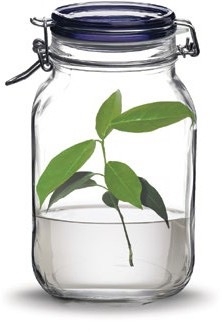